|
 |
STAR focus: Observation of the Antimatter Hypernucleus $^4_{\bar{\Lambda}}\overline{\hbox{H}}$
|
Matter-antimatter asymmetry is a research topic of fundamental interest, as it is the basis for the observed dominance of matter over antimatter in the universe. High energy nuclear collisions create conditions similar to the Universe microseconds after the Big Bang, with comparable amounts of matter and antimatter. Much of the antimatter created escapes the rapidly expanding fireball without annihilation, making such collisions an effective experimental tool to create heavy antimatter nuclear objects and study their properties.
In this work, we report the first observation of the antimatter hypernucleus $^4_{\bar{\Lambda}}\overline{\hbox{H}}$, composed of a Λ, an antiproton and two antineutrons. This discovery was made through its two-body decay channel $^4_{\bar{\Lambda}}\overline{\hbox{H}} {\rightarrow}^4\overline{\hbox{He}}+\pi^+$ in the STAR experiment at the Relativistic Heavy Ion Collider.
We use Kalman Filter algorithm to analyse the data totaling 6.6 billion collision events in the Au+Au, Ru+Ru, and Zr+Zr collisions with the collision energy $\sqrt{s_{NN}}=200$GeV, as well as U+U collisions with the collision energy of $\sqrt{s_{NN}}=193$GeV. We obtained 15.6 signal candidates for the antimatter hypernucleus ${}^{4}_{\bar{\Lambda}}\overline{\hbox{H}}$, with the estimated background counts 6.4 and significance of 4.7.
We also obtained 941 ${}^{3}_{\Lambda}\hbox{H}$ signal candidates, 637 ${}^{3}_{\bar{\Lambda}}\overline{\hbox{H}}$ signal candidates and 24.4 $^4_\Lambda\hbox{H}$ signal candidates using the same method.
|
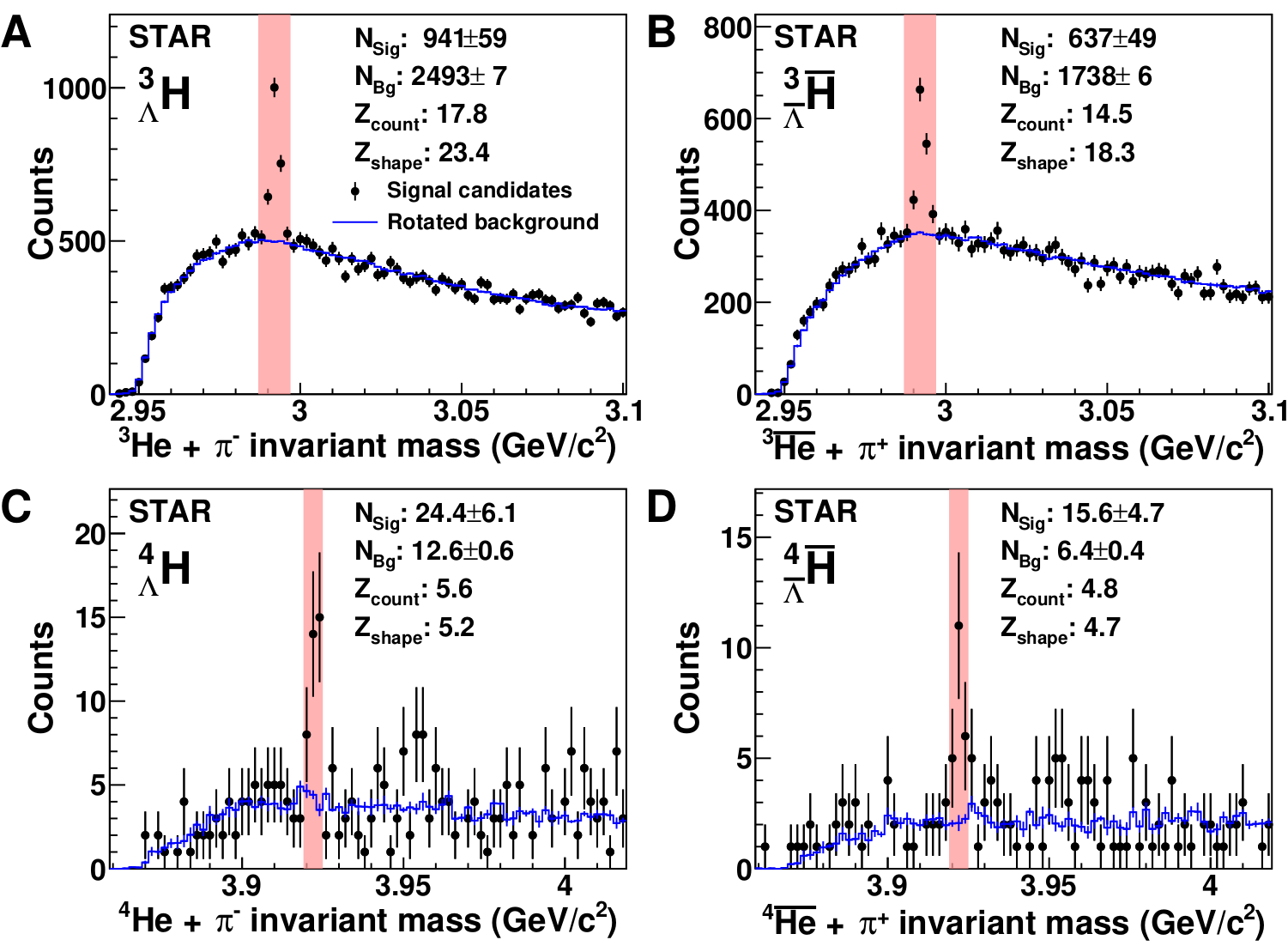 |
Figure: Invariant-mass distributions of $^3\hbox{He}+\pi^-$ (A), $^3\overline{\hbox{He}}+\pi^+$ (B), $^4\hbox{He}+\pi^-$ (C) and $^4\overline{\hbox{He}}+\pi^+$ (D).} The solid bands mark the signal invariant-mass regions. The obtained signal count ($N_{\rm Sig}$), background count ($N_{\rm Bg}$), and signal significances ($Z_{\rm count}$ and $Z_{\rm shape}$) are listed in each panel.
|
Comparing lifetimes between a particle and its corrsponding antiparticle is an important experimental way to test the $CPT$ symmetry and to search for new mechanisms that cause this matter and antimatter asymmetry. In this work, we measured the lifetimes of ${}^{3}_{\Lambda}\hbox{H}$, ${}^{3}_{\bar{\Lambda}}\overline{\hbox{H}}$, ${}^{4}_{\Lambda}\hbox{H}$, and ${}^{4}_{\bar{\Lambda}}\overline{\hbox{H}}$. The lifetime difference between the hypernuclei and antihypernuclei was compared and we haven't found the CPT symmetry broken within the measurement uncertainty.
Various production yield ratios among (anti)hypernuclei and (anti)nuclei are measured. We found $\left(^3\overline{\hbox{He}}/^3\hbox{He}\right)\times\left(\bar{p}/p\right)\approx ^4\overline{\hbox{He}}/^4\hbox{He}$ and $\left(^3_{\bar{\Lambda}}\overline{\hbox{H}}/^3_{\Lambda}\hbox{H}\right)\times\left(\bar{p}/p\right) \approx ^4_{\bar{\Lambda}}\overline{\hbox{H}}/^4_{\Lambda}\hbox{H}$, these agree with the thermal model and coalescence picture predictions. And $^4_{\Lambda}\hbox{H}/^4\hbox{He}$ and $^4_{\bar{\Lambda}}\overline{\hbox{H}}/^4\overline{\hbox{He}}$ is expected to be about 4 times higher than $^3_{\Lambda}\hbox{H}/^3\hbox{He}$ and $^3_{\bar{\Lambda}}\overline{\hbox{H}}/^3\overline{\hbox{He}}$, respectively, hinting that $^4_{\Lambda}\hbox{H}$ has an excited state with spin 1. These measurements shed light on their production mechanism.
This work has been published by NATURE in August 2024.
Posted Aug 26, 2024
Previous STAR Focus Features
|
 |
STAR focus: Measurements of charged-particle multiplicity dependence of higher-order net-proton cumulants in pโฏ+โฏp collisions at $\sqrt{s} = 200$ GeV from STAR at RHIC
|
One of the ultimate goals of heavy-ion collision experiments is to map the phase diagram of nuclear matter, the so-called QCD phase diagram, with respect to temperature (T) and baryon chemical potential ($ยต_{B}$). Higher-order cumulants of net-baryon multiplicity distributions are expected to be sensitive to the QCD phase structure. The STAR experiment observed that the $6^{th}$-order fluctuation of the net-proton multiplicity distribution becomes progressively negative systematically from peripheral to central Au+Au 200 GeV collisions, which hints at a smooth crossover transition at $ยต_{B}$=25 MeV.
Recently, the STAR experiment published the net-proton higher-order cumulants from p+p 200 GeV collisions. Experimental results from the LHC, such as collectivity and strangeness enhancement, suggest that the Quark Gluon Plasma (QGP) could be formed in high-multiplicity p+p collisions, although it does not necessarily indicate that thermalized QCD drops of matter have been created. This can be tested by higher-order correlations in p+p collisions at top RHIC energy and LHC energies.
The figure below (Phys.Lett.B 857 (2024) 138966 ) shows the charged-particle multiplicity dependence of the cumulant ratios, (a) $C_4$/$C_2$, (b) $C_5$/$C_1$, and (c) $C_6$/$C_2$ of net-proton multiplicity distributions. Results from p+p 200 GeV minimum bias collisions are close to those from peripheral Au+Au 200 GeV collisions. On the other hand, the ratios measured at each charged-particle multiplicity bin in p+p collisions decrease with increasing the multiplicity more rapidly than Au+Au collisions, and $C_5$/$C_1$ and $C_6$/$C_2$ values reach zero at the highest multiplicity bin. The results favor the negative values of the ratios at higher multiplicity events. Although part of the decrease as a function of the charged particle multiplicity in a given event is due to baryon conservation, the negative ratios in the context of lattice QCD calculations imply that even in the small ๐+๐ system, thermalized QCD matter may be created in the highest-multiplicity collisions. Further, the steeper slope indicates that thermalized QCD matter is created more efficiently in high multiplicity ๐+๐ collisions than that in heavy-ion collisions. Systematic measurements of the cumulant ratios from higher collision energies and in larger colliding systems will provide important information on the underlying dynamics of thermalization in high-energy collisions.
|
|
Figure: Net-proton cumulant ratios, (a) $๐ถ_{4}$โ$๐ถ_{2}$, (b) $๐ถ_{5}$โ$๐ถ_{1}$, and (c) $๐ถ_{6}$โ$๐ถ_{2}$ as a function of charged-particle multiplicity for ๐+๐ collisions and Au+Au collisions at 200GeV. Cyan markers represent event averages from the ๐+๐ collisions. Results from Au+Au collisions are shown as triangles. Red and grey bands show the systematic uncertainties for ๐+๐ collisions and Au+Au collisions, respectively. The Skellam baselines are shown in dotted lines. The purple bands show corresponding susceptibility ratios of baryon number from lattice QCD calculations.
|
Posted Oct 22, 2024
Previous STAR Focus Features
|
 |
STAR focus: Exclusive J/ฯ, ฯ(2s), and e+eโ pair production in Au+Au ultra-peripheral collisions at RHIC
|
Recently, we measured exclusive J/ฯ, ฯ(2s), and di-electron pair photoproduction in Au+Au ultra-peripheral collisions at RHIC using the STAR detector. For J/ฯ production, both coherent (nucleus remains intact) and incoherent (nucleus breaks up) processes were observed. For the first time, it was found that both coherent and incoherent J/ฯ production are suppressed in gold nuclei compared to a free proton, with the suppression being even stronger for the incoherent process. Given the RHIC kinematics, gluon saturation is not expected to play a significant role, so the observed large nuclear suppression suggests additional effects, such as nuclear shadowing. Indeed, the Leading Twist Approximation nuclear shadowing model provides a better fit to the data, particularly for coherent J/ฯ production. This research is focused on the intermediate energy range in heavy-ion UPCs, which is sensitive to the transition between regions dominated by dense gluons and those by valence quarks.
|
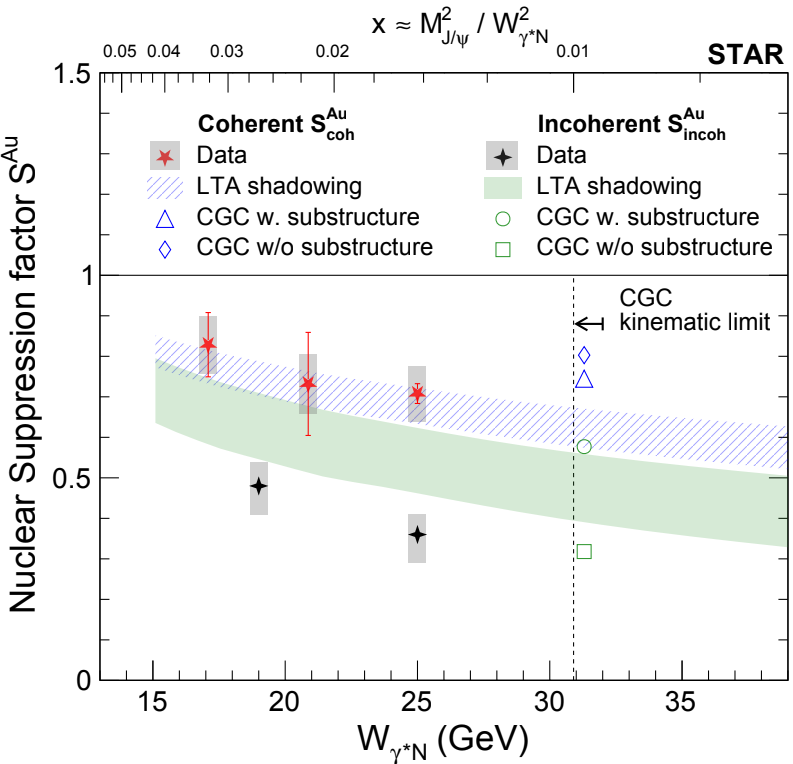 |
|
Figure:Nuclear suppression factor of coherent and incoherent J/ฯ photoproduction in Au+Au UPCs. The data are compared with the nuclear shadowing model and the CGC model.
Additionally, we have made the first cross-section measurement of ฯ(2s) photoproduction at RHIC and found that the ratio between ฯ(2S) and J/ฯ is consistent with free proton data, indicating that the nuclear suppression does not significantly differ between these two vector mesons. Finally, we reported di-electron photoproduction via the Breit-Wheeler process, extending to high invariant mass regions up to 6 GeV, which supports the nuclear breakup model involving forward neutron emission and associated photon fluxes.
In summary, these UPC measurements of vector mesons and dileptons provide valuable insights into the inner structure of nucleons and nuclei, helping to pave the way for the upcoming electron-ion collider.
|
Posted Oct 30, 2024
Previous STAR Focus Features
|
 |
STAR focus: Imaging shapes of atomic nuclei in high-energy nuclear collisions
|
The STAR Collaboration recently published "Imaging Shapes of Atomic Nuclei in High-Energy Nuclear Collisions" in Nature.
This work has been selected in Nature News & Views, highlighted in an interview for Nature Podcast, and has been selected in other Nature NEWS. Atomic nuclei, composed of protons and neutrons, are self-organized, many-body quantum systems bound by strong-nuclear forces within femtometer-scale space. Revealing the shape of nuclei has been a scientific pursuit for over a century, beginning with Rutherford's scattering experiments. Numerous models, including the Fermi gas model, Liquid drop model, Shell model, and Collective model, have been developed to study nuclear structure, with two of these models earning Nobel Prizes. Currently, our understanding of nuclear shape largely comes from low-energy spectroscopic and scattering experiments. Investigating nuclear shape across the nuclear chart has been an important area of research and is crucial for topics such as nucleosynthesis, nuclear fission, and neutrinoless double beta decay ($0 \nu \beta \beta$).
A pertinent question arises: How do shapes observed in high-energy colliders compare to those derived from low-energy experiments? High-energy nuclear collisions, an utterly destructive process, have a much shorter timescale than nuclear rotational degrees of freedom, allowing access to many-body nucleon distribution within each nucleus. Head-on (near-zero impact parameter) collisions of prolate deformed nuclei present two extreme configurations, body-body (top) and tip-tip (bottom) collisions. The collision of two Lorentz-contracted pancake-like shapes of nuclei at high energy can generate a 3D profile of the initially produced quark-gluon plasma. The shape and size of the initial overlap region, as well as those of the final fireball after hydrodynamic expansion, differ significantly between these two collision configurations, as shown in the figure.
|
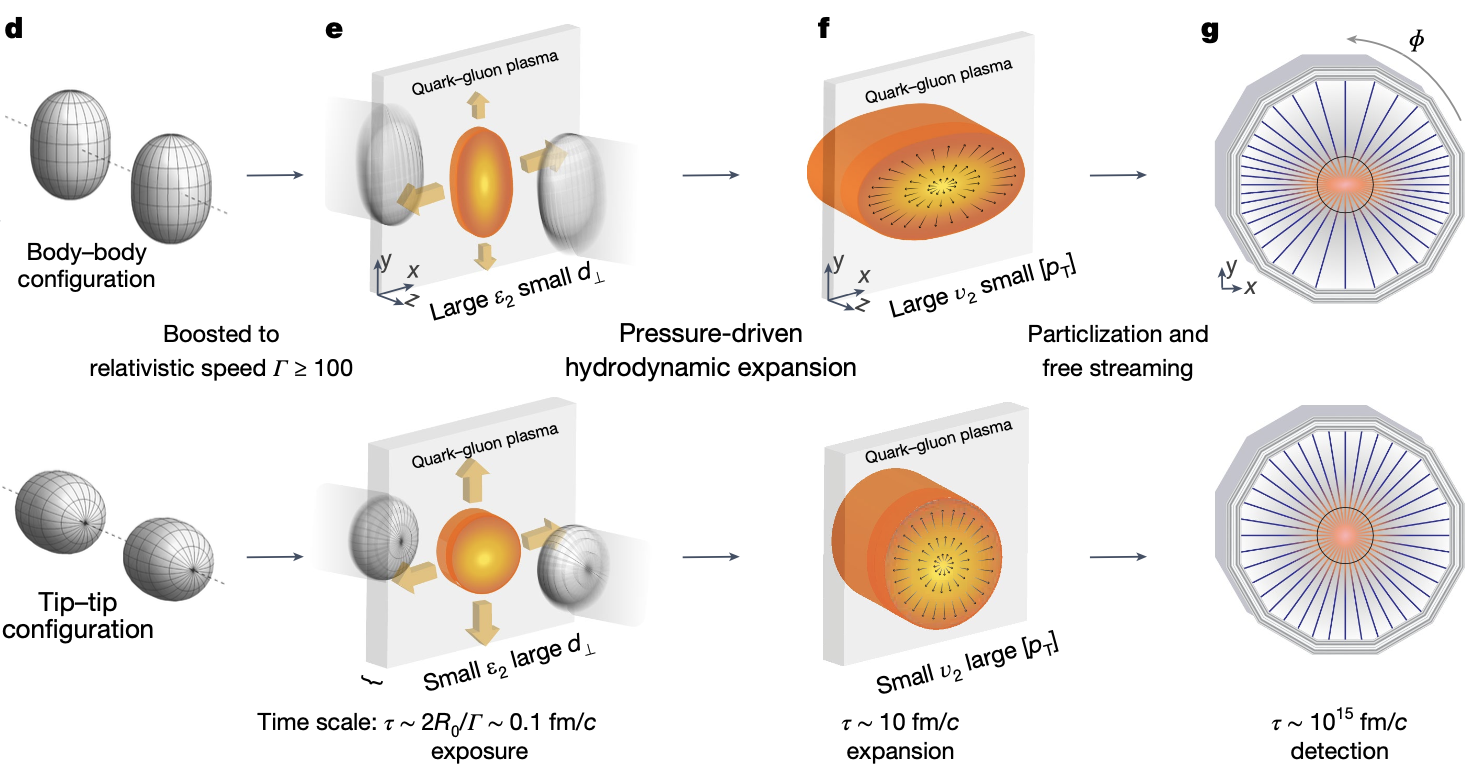 |
|
In this article, the STAR Collaboration developed a new method, "collective flow assisted nuclear shape imaging" method, which images the nuclear global shape by colliding them at ultrarelativistic speeds and analyzing the collective response of outgoing debris. This technique captures a collision-specific snapshot of the spatial matter distribution within the nuclei, which, through the hydrodynamic expansion, imprints patterns onto the particle momentum distribution observed in detectors. By taking ratios of the nearly equal mass number $^{238}$U+$^{238}$U and $^{197}$Au+$^{197}$Au collisions, final state effects are almost completely canceled, leaving model uncertainties primarily from initial conditions. Comparisons between data and IP-Glasma+MUSIC model delineate preferred $\beta_{2 \mathrm{U}}$ ranges, yielding $\beta_{2 \mathrm{U}}=0.297 \pm 0.015$ and $\gamma_{\mathrm{U}}=8.5^{\circ} \pm 4.8^{\circ}$ (mean and one standard deviation). Corresponding constraints from Trajectum are $\beta_{2{\mathrm{U}}}=0.275 \pm 0.017$ and $\gamma_{\mathrm{U}}=15.5^{\circ} \pm 7.8^{\circ}$. Combining constraints from both models yields $\beta_{2 \mathrm{U}}=0.286 \pm 0.025$ and $\gamma_{\mathrm{U}}=8.7^{\circ} \pm 4.5^{\circ}$. These values are broadly consistent with previous extractions based on low energy data.
Our approach offers a novel method for imaging nuclear shapes, enhances understanding of the initial conditions in high-energy collisions, and addresses the critical issue of nuclear structure evolution across energy scales. Future research could leverage colliders to conduct experiments with selected isobaric or isobar-like pairs, further enabling interdisciplinary research in nuclear physics.
|
Posted Nov. 6, 2024
Previous STAR Focus Features
|
|
|
|
code of conduct
|
The STAR Collaboration
believes that our scientific
mission is best achieved by...
Read More
|
community links
|
|
collaboration links
|
Upcoming events
2025 RHIC/AGS Annual Users' Meeting May 20 - 23, 2025, BNL
Past events
STAR Pre-QM25 Meeting April 2 - 5, 2025, Frankfurt Institute for Advanced Studies (FIAS)
STAR Collaboration Meeting Spring 2025 March 2 - 7, 2025, BNL
STAR eTOF and BES-II Workshop Dec. 3 - 6, 2024, BNL
STAR Collaboration Meeting Fall 2024 Oct. 21 - 25, 2024, WUT Warsaw
STAR Analysis Meeting Sep. 09 - 11, 2024, Online
ALICE-STAR India Collaboration Meeting June 24 - 27, 2024, Institute of Physics Bhubaneswar
STAR Regional Meeting 2024 May 27 - 29, 2024, Czech Technical University in Prague
STAR Collaboration Meeting Spring 2024 March 18 - 22, 2024, BNL
STAR eTOF Workshop Feb 19 - 20, 2024, GSI
STAR Analysis Meeting Jan. 16 - 18, 2024, Online
ALICE-STAR India Collaboration Meeting Nov. 21 - 24, 2024, Institute of Physics Bhubaneswar
Meeting Archive
STAR Computer Account
Experiment status |
Shift signup
Training and guest registration
STAR Juniors Materials
Submit papers, talks, meetings, news, errors, ideas, ...
|
|
recent news
|
December 11, 2024
Please join us in congratulating Dr. Diptanil "Neil" Roy from Rutgers
University, who successfully defended his Ph.D. thesis, "Tracing Charm
Through Quark Gluon Plasma Using Jet Tomography.โ His advisor was Prof.
Sevil Salur.
Neil will continue in industry, and we wish him all the best in his future
career!
Congratulations, Dr. Roy, and many thanks, Sevil, for your continued
support in mentoring the next generation of physicists.
October 25, 2024
Please join us in congratulating Dr. Xinyue Ju and Dr. Fan Si, both from
USTC, who, on October 25, successfully defended their Ph.D. theses
- Xinyue Ju on "Measurement of D± meson production and the application
of the Kalman Filter Particle method in Au+Au collisions at RHIC", with
co-supervisors Profs. Xin Dong and Yifei Zhang
- Fan Si on "Study of proton number fluctuations in heavy-ion collisions at
RHIC", with co-supervisors Profs. Nu Xu, ShinIchi Esumi, and Yifei
Zhang
Fan and Xinyue will continue to work in our field.
Congratulations, Dr. Ju and Dr. Si!
Many thanks to Yifei, Xin, Nu, and ShinIchi for your continued support in
mentoring the next generation of physicists.
October 23, 2024
Please join us in congratulating Dr. Xin Wu from USTC, who, last Friday,
successfully defended his Ph.D. thesis, "Proton-antiproton photoproduction
in relativistic heavy-ion collisions.โ His co-advisors were Profs.
Wangmei Zha and Zebo Tang.
Xin will continue as a postdoc in our field.
Congratulations, Dr. Wu, and many thanks, Wangmei and Zebo, for your
continued support in mentoring the next generation of physicists.
|